Electrochemical Cell
Electrochemical Cell
Table of Contents
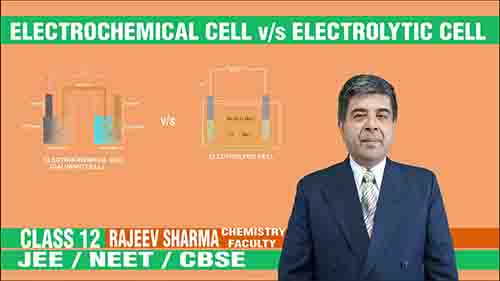
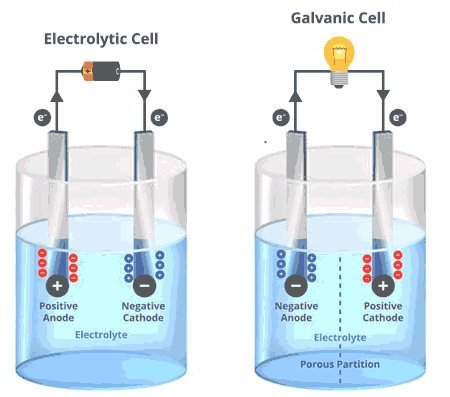
Introduction
In the fascinating realm of chemistry, electrochemical cells play a pivotal role in converting chemical energy into electrical energy and vice versa.
Whether powering your favorite gadgets or facilitating essential biochemical processes, understanding these cells is critical for students and enthusiasts alike.
This blog post delves into the two primary types of electrochemical cells: galvanic cells, which harness spontaneous reactions to generate electricity, and electrolytic cells, which utilize external energy to drive non-spontaneous reactions.
Join us as we explore the principles behind these cells, their unique characteristics, and their real-world applications, making complex concepts accessible for everyone eager to deepen their knowledge of electrochemistry.
Difference between Galvanic & Electrolytic cell
Introduction to Electrochemical Cells
In the world of chemistry, electrochemical cells play a vital role in converting chemical energy into electrical energy and vice versa.
As an essential component of numerous applications—from batteries to electrolysis—understanding their fundamentals is crucial for students and professionals alike. In this post, we will explore the two primary types of electrochemical cells: Galvanic cells and Electrolytic cells.
What is an Electrochemical Cell?
Before diving into the specifics of Galvanic and Electrolytic cells, let’s define what an electrochemical cell is. An electrochemical cell consists of two electrodes—an anode and a cathode—immersed in an electrolyte solution, with a flow of electrons between the electrodes. This movement enables chemical reactions that can generate or store electrical energy.
1. Galvanic Cells: Power from Spontaneous Reactions
Definition and Functionality
Galvanic cells, also known as voltaic cells, are designed to convert chemical energy from spontaneous redox reactions into electrical energy. These cells generate electricity through the spontaneous reaction of the reactants (oxidation and reduction) with no external power source needed.
Typical Structure
A Galvanic cell typically consists of two half-cells connected by a salt bridge that allows for ion flow. Each half-cell contains an electrolyte solution and electrodes made of different metals.
Common Examples
Common examples of Galvanic cells include zinc-carbon batteries and alkaline batteries. These cells are widely used in everyday appliances, showcasing their practicality in energy conversion.
2. Electrolytic Cells:
Driving Non-spontaneous Reactions
Definition and Functionality
In contrast to Galvanic cells, electrolytic cells use external electrical energy to drive non-spontaneous chemical reactions.
This process can be used for tasks such as electrolysis, where electrical energy is applied to separate elements from their compounds.
Typical Structure
Electrolytic cells are similar in structure to Galvanic cells, with anode and cathode, but require an external power source, which often takes the form of a DC power supply to facilitate electrolysis.
Common Examples
Electrolytic cells are commonly found in industrial applications such as electroplating, metal extraction, and water splitting to produce hydrogen fuel.
Key Differences Between Galvanic and Electrolytic Cells
While both Galvanic and electrolytic cells share similarities in construction, their operational principles and applications differ significantly.
Spontaneity: Galvanic cells operate spontaneously; electrolytic cells require electrical energy input.
Energy Conversion: Galvanic cells convert chemical energy into electrical energy, while electrolytic cells do the reverse.
Applications: Galvanic cells are used in batteries; electrolytic cells are essential in processes like metal plating and chemical synthesis.
Standard Hydrogen Electrode (SHE)
The Standard Hydrogen Electrode (SHE) serves as the universal reference point for measuring electrode potentials in electrochemical cells. This fundamental component plays a crucial role in the field of electrochemistry, providing a baseline against which other half-cell potentials can be compared.
The SHE consists of a platinum electrode immersed in a solution of hydrogen ions (H⁺), typically 1 molar sulphuric acid (H₂SO₄), where hydrogen gas (H₂) is bubbled over the electrode surface at a pressure of 1 atmosphere. One of the defining features of the SHE is its standardized potential, which is set at 0.00 volts under standard conditions 25°C and 1 atm pressure.
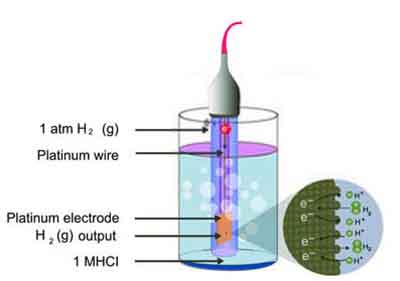
This makes it an invaluable tool for researchers and students alike who are exploring the electrochemical properties and reactions of various substances. By using the SHE as a reference, it becomes easier to characterize the behavior of other electrochemical cells, such as Galvanic cells, which convert chemical energy into electrical energy.
When discussing electrochemical cells, it’s essential to understand the role of the Standard Hydrogen Electrode in the context of redox reactions. The SHE represents both the oxidation and reduction half-reactions of hydrogen, allowing for a clear illustration of how potentials are calculated.
For example, when paired with a different half-cell, the potential difference can be determined, which is critical in predicting the direction of electron flow and the feasibility of a reaction. In summary, the Standard Hydrogen Electrode is not merely a theoretical construct; it is a practical and foundational element in electrochemistry that facilitates the understanding and development of various types of electrochemical cells, including the Galvanic cells and other cells utilized in energy storage, sensors, and batteries.
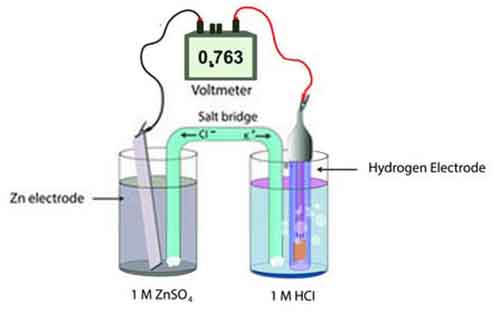
Cell Representation
Understanding the representation of electrochemical cells is a crucial aspect of mastering electrochemistry, particularly for Class 12 students preparing for the CBSE board exam. The standard representation of an electrochemical cell typically adheres to the notation outlined by the IUPAC conventions, which helps in clearly delineating the components of the cell and their functions.
An electrochemical cell is represented as a sketch consisting of two half-cells, each situated in its own container. The anode (negative electrode) is represented on the left, while the cathode (positive electrode) occupies the right side.
The representation follows a specific format:
Anode | Anionic Solution || Cationic Solution | Cathode
For example, let’s consider a galvanic cell composed of zinc and copper electrodes. The representation would be written as:
Zn(s) | Zn²⁺(aq) || Cu²⁺(aq) | Cu(s)
In this notation: – Zn(s) indicates solid zinc at the anode, oxidized to Zn²⁺ ions. Zn²⁺(aq) is the aqueous solution of zinc ions, maintaining the half-cell reaction on the left. –
|| denotes the salt bridge or porous membrane separating the two half-cells, which facilitates ion flow.
Cu²⁺(aq) represents the aqueous solution of copper ions at the cathode, where reduction occurs. Cu(s) designates solid copper, demonstrating the deposition during the reduction reaction.
This clear delineation helps students not only visualize but also understand the flow of electrons and ions during the electrochemical processes. Additionally, knowing how to represent the cell properly can aid in analyzing the overall cell potential and combining half-reactions effectively critical skills for excelling in your board exams.
Configure your study sessions with visual aids and practice questions surrounding cell representations to reinforce this essential concept in electrochemistry!
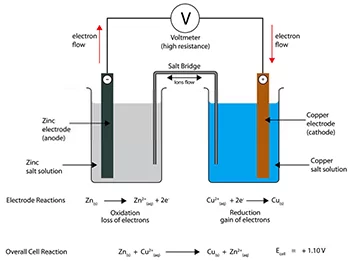